Lighting for Health: Human-Centric Lighting
Overview
Light—from the sun and artificial light sources—profoundly effects our body’s circadian system. Light is a key factor in human productivity, alertness, sleep quality, and health. And, as we are learning, “poor” lighting can have unfavorable effects on health and well-being. A lighting revolution is happening as awareness of human-centric lighting (HCL) has emerged in recent years.
The HCL approach focuses on designing lighting for workplaces, public spaces, and homes that support circadian function. HCL lighting schemes can be challenging to achieve indoors, however. In the daytime, cool blue-toned light (e.g.,5000 K) helps with alertness and wakefulness; in the evenings, warm light with an orange spectrum (e.g., 2700 K) helps our bodies wind down for a good night’s sleep.
LED lighting offers many benefits, including low power use and long bulb life. However, the color profile of most standard LEDs makes it difficult to strike the right balance of color tone and brightness to provide a comfortable and productive office or work environment.
How can architects, lighting designers, and building owners balance the need for artificial illumination indoors and at night with concerns for human well-being and productivity? The answer is human-centric lighting (HCL), a new, holistic approach developed in the last decade. HCL encompasses the science of circadian lighting to mimic the effect on our biological clock of natural daytime/nighttime light cycles, along with broader considerations building occupants’ productivity, mood, and comfort.
Newly developed LED technology now enables lighting schemes that better support human circadian function. Breakthrough products such as the Luminus Salud™ LED are helping designers meet new Well Building Standards and achieve HCL designs that balance functional and circadian factors.
This White Paper Explores:
- Why light quality profoundly affects humans and how electrical lighting can cause health problems and impact productivity
- Circadian lighting and the new science of human-centric lighting
- How to measure and define the characteristics of light, such as color temperature(CCT) and brightness (illuminance, or lux)
- The ideal lighting characteristics for human activity, safety, and health
- How to apply human-centric approaches to lighting design schemes
- LED technology that makes HCL possible and new solutions to optimize lighting for human activity
The Challenge
Where would we be without electric light? Much of modern life revolves around artificial lighting. It cheers us during the long dark days of mid-winter. It brings safety to our streets and extends not only our workdays but our ability to enjoy our after-work hours as we read, relax, enjoy entertainment and sports, and socialize.
Do we know what effect all of this artificial light might be having on human health, productivity, and well-being? Each year more studies suggest possible connections between “bad” light and cancer, diabetes, obesity, sleep disorders, and mental health issues. Overly bright of the wrong “color” lighting can disrupt our circadian cycles of sleep and wakefulness. Electronic devices, such as the computer screens many of us spend our days staring at and the smartphones we check as we settle in at home may emit light that can disturb sleep.
In addition to potential health effects, poor sleep quality and sleep deprivation can lead to safety issues. Intermittent sleep impairs a person’s ability to function as much as having a blood-alcohol level of 0.08. Multiple health issues and accidents, including as many as 25 percent of all automobile accidents [1], are related to lack of sleep—a lack of sleep that can be caused by the wrong lighting.
The Solution
But there is a solution. We now understand how lighting affects human biology and how to design lighting schemes that support our health and functioning. By leveraging lighting products such as LEDs (light-emitting diodes) that optimize the right colors and aspects of the visible light spectrum, we can continue to enjoy the benefits of artificial illumination, day or night, without adverse consequences to our health and safety.
Luminus Products for Human-Centric Lighting Designs
Dynamic Chip-on-Board (COB) Modules
- CCT-Tunable COB LEDs: These modules offer a choice of 9, 14, 18, and 22mm LES(light emitting surface) options, all with two independent channels (warm and cool)to deliver CCTs ranging from 6500K to 2700K or from 4000K to 1800K. This wide range of options provides luminaire makers with flexibility in designing human-centric lighting applications with independent CCT and dimming control. LEARN MORE
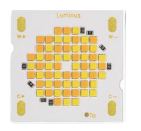
- Luminus Warm Dimming COB LEDs: Luminus Warm Dimming Modules replicate the behavior of traditional bulb technology by dynamically changing CCT from 3000K to1800K or from 4000K to 2700K as they are dimmed. This “dim-to-warm” effect provides a relaxing, human-centric environment, ideal in restaurants, bars, hotels, retail, and residential environments. LEARN MORE
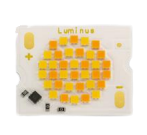
PerfectWhite™
Engineered to achieve a superior white color spectrum illumination, PerfectWhite provides a uniform spectral response (no cyan dip) and no UV or violet emissions. Ideal for museums and art galleries, specialty retailers, and hospitality venues. LEARN MORE
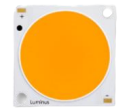
Salud™
Salud LEDs are specified for melanopic/photopic ratios (MRs) in addition to CRI, R9, CCT, and Flux. By enhancing the spectrum with cyan for melatonin suppression and enriching the660nm emission for natural skin tones, Luminus Salud LEDs can deliver warmer, more comfortable light that keeps people healthy, alert, and productive. LEARN MORE
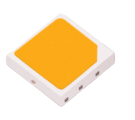
Background
Light has a tremendous, often subconscious, impact on our moods, health, and productivity. It's not surprising—almost every living thing on Earth was shaped in some way by the sun over many eons(Figure 1). All species, including humans, have evolved as our planet revolves around the sun.
With each revolution, natural human productivity responded to fluctuations in the length of days. Humans have further adapted over millennia to the 24-hour cycle of day and night as the Earth spins on its axis. We are naturally diurnal creatures, active during the day and relaxing and sleeping at night. This adaptation is what’s known as the circadian cycle.
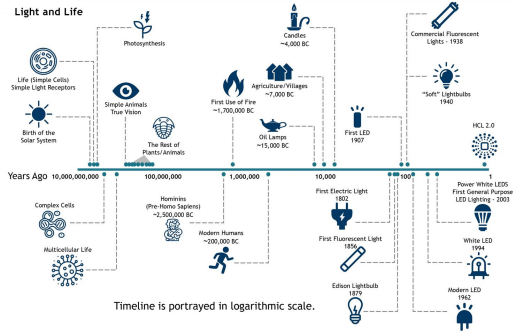
However, today we have more light at night than ever before in history. For the past 150 years, we have come to work, live, and play under artificial illumination at all hours. Added light boost people’s moods and increases productivity. Artificial illumination contributes to the 24/7 economy, where factories can function day and night, and illuminated streets increase safety. People can experience light “as bright as day” at any hour, staying active throughout the night.
But all this increased productivity comes with some downsides. Office workers frequently complain of tiredness during the day when sitting inside under fluorescent or other non-natural light. Our artificial light and the light emanating from our screen devices is draining us and keeping us awake.
The U.S. Centers for Disease Control and Prevention says that exposure in the evening hours to blue light and white light, which contains the color blue, can disrupt and prevent sleep [2]. Blue light exposure upsets circadian rhythms by suppressing our body’s release of melatonin, a sleep hormone.
The Dangers of Sleeplessness
Sleep concerns more than just personal comfort. Sleep promotes healing, fighting infections, and forming memories from the day’s experiences. Sleep also regulates insulin. A lack of sleep disrupts the insulin and glucose system, making individuals prone to diabetes. Sleep deprivation can increase the body’s inflammatory response, limit the functions of cancer fighting cells and the formation of antibodies to fight infection.
Moreover, fatigue limits our ability to think, learn, and make decisions. A lack of sleep can lower inhibitions, making people prone to risky behaviors, such as driving under the influence of alcohol. Even without mind-altering substances, driving with less than four hours of sleep or after intermittent sleep has the same harmful effects on alertness, reflexes, and judgment as a blood alcohol level of 0.08.
Clearly, sleep deprivation presents serious issues for industrial society. Given the critical effect that light has on human sleep patterns and sleep quality, the first step to formulating an optimal lighting solution is understanding how we see light.
Human Vision and Response to Light
The human eye contains light receptors called rods and cones, as shown in Figure 2. The rods enable us to see in low-light conditions with limited or no color perception, referred to as scotopic vision. Thus dusk and nighttime scenes appear to us in black and white. The cones are responsible for our daytime light sensing (photopic) and color perception.
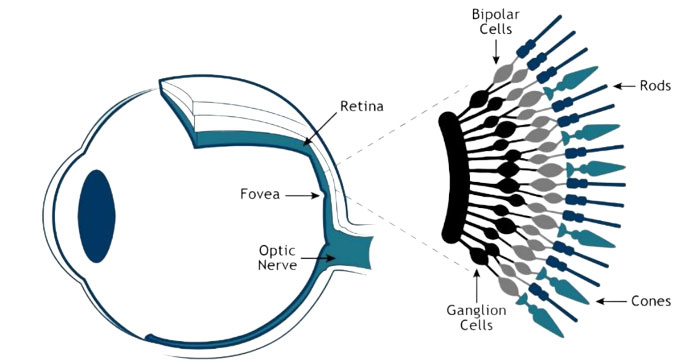
We see because light strikes the rods and cones of the retina, which fires the proteins rhodopsin and photopsin. This instantaneous chemical change sends signals via the optic nerve to the brain. The brain then processes those signals as images.
In the past 20 years, researchers have discovered other photosensitive cells in the eye—cells not involved in visual processing. In the retina, scattered above the color- and shadow-sensitive rods and cones are the retinal ganglion cells (RCG), also called photosensitive retinal ganglion cells (ipRGCs).The ipRGCs form the main neural pathway to the brain’s hypothalamus region, the location of our circadian clock, the mechanism that aligns our internal body cycles—our circadian rhythms.
To align those rhythms, as light enters the eyes it fires a photosensitive protein called melanopsin in each ipRGC. In a multistep process, melanopsin suppresses the sleep-inducing hormone melatonin during the day and gradually ramps up melatonin production as light fades into night (Figure 3). Why? Because melanopsin responds most to the primary colors of daylight—blue and cyan. Thus, the human eye responds to light as a visual perception stimulus, but also triggers our bodies to respond hormonally by releasing melatonin. What’s more, the RGCs control circadian effects even in the visually impaired.
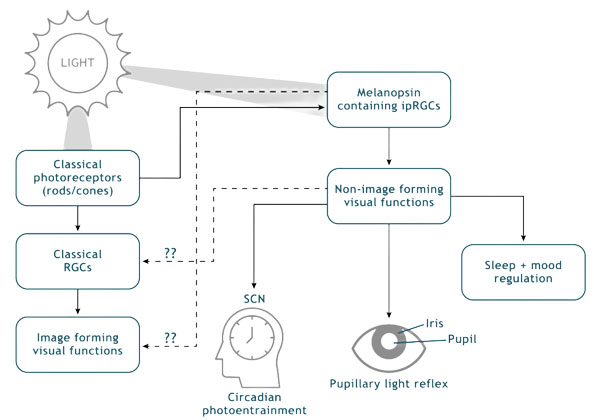
CASE STUDY: Light Fatigue
A 47-yr-old male complaining of severe fatigue and daytime sleepiness was admitted to the Institute for Fatigue and Sleep Medicine. [3]
Over 20 years, he had experienced irregular sleep-wake patterns, depression, and chronic fatigue symptoms that had worsened over time to the point that it impaired his ability to function from day to day. He had to cut back to working only part-time as a result. Treatment with antidepressant drugs and melatonin thus far had failed to provide relief.
But as his clinical team investigated further, they learned that the man had worked the late afternoon/night shifts. Most significantly, he worked steadily under a daylight-intensity lamp—professional diamond-grading equipment that produced more than 8000 lux. This light would also likely have had a high blue CCT.
With treatment that included exposure to real morning light, known as bright-light therapy, and taking melatonin supplements in the evening, the patient was able to experience “profound improvement in maintaining wakefulness during the day” [3] and healthy sleep at night.
This case demonstrates the potential dangers of bright light exposure at night to human circadian rhythms and sleep-wake cycles, and the risk of depression and melancholy. Not everyone sits under diamond-grading lamps, but even blue/white light emanating from a normal cell phone can disrupt human sleep.
About Light
What do we know about the quality of the artificial electrical light in our offices, schools, or homes? How does it compare to the natural light conditions and cycles that support our body’s natural rhythms? To understand what constitutes “healthy” light, we start by looking at how light is characterized and quantified. Most people think of light in terms of what we see, but visible light is just a sliver of the full spectrum of electromagnetic radiation. Experts describe this radiation by it senergy or, inversely, by its wavelength, as shown in Figure 4.
The electromagnetic spectrum extends from short wavelength, high energy gamma rays on one end all the way to long wavelength, low energy microwaves and radio waves on the other. A small band in the middle of the spectrum is the range of light visible to the human eye. Visible light has wavelengths within the range of approximately 380 nanometers (nm) to 750 nm, bounded by ultraviolet (UV) at the low end of the range and infrared (IR) at the high end.

Quantifying Light
The amount of light emitted from or reflected off an object is called its luminance—commonly referred to as brightness. Luminous flux is a measurement of the total amount of light a light source emits, integrated over the entire angular span of the light. We quantify luminous flux in units of lumens (lm), a photometric unit of measurement. Luminous intensity is a measure of the light that shines from the source in a given direction. Illuminance refers to the amount of light that shines on to a surface, measured in lumens per square meter (lm/m2), also called lux (refer to Figure 5). Lux is an essential consideration for interior lighting design for spaces where humans live and work.

Quantifying Color
In 1931, the CIE (Commission Internationale de L’Eclairage, International Commission on Illumination) defined a standard for scientifically quantifying the physical properties of colors. The CIE color space describes the human eye’s response to visible light and our perception of color for distributions of wavelengths. Since its inception, the CIE color space (Figure 6) has enabled accurate measurement, representation, and replication of these colors for a vast range of applications such as printing, light sources, and illuminated display screens.
The black-body locus (also called the Planckian locus) shown in Figure 6 is a plot of the chromaticity coordinates of light sources known as black-body radiators. You can conceptualize a black-body radiator as a platinum sphere held at a specific temperature Kelvin (K). The black-body curve is shown within the CIE diagram to indicate the points where the light appears white. Artificial white light sources have color coordinates near the black-body curve. But white light spectral power distributions (SPDs) can include many combinations of wavelengths rather than the smooth curves associated with a black-body source.
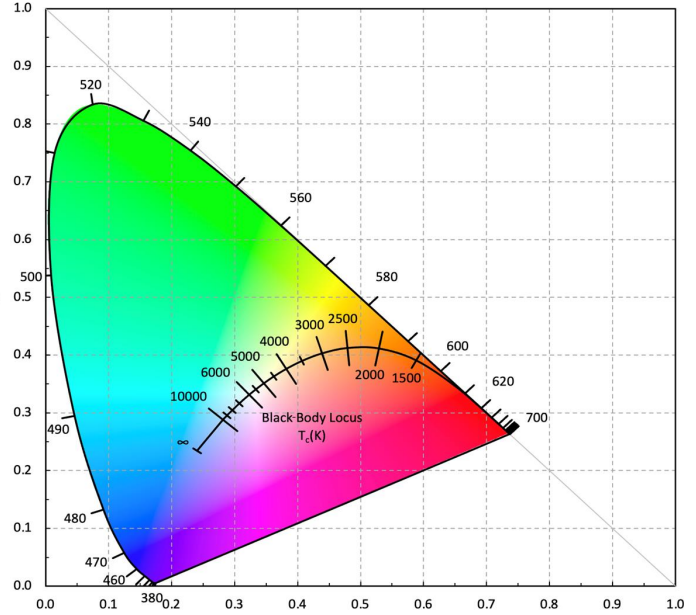
Correlated Color Temperature.
Correlated color temperature (CCT) is a way to characterize the color appearance of any white light source with a single number. Artificial white light can be produced using combinations of all the colors in the visible spectrum. Different amounts of each wavelength will cause the light to appear “cooler” (more blue/cyan wavelengths) or “warmer” (more yellow/orange wavelengths).
The CCT of a light source is the point on the Planckian locus that matches most closely (perceptually)to the chromaticity coordinates of the light—called its color temperature. If a light source is not a black-body radiator, but its chromaticity coordinates lie close to the Planckian locus, we can characterize its color by its CCT. CCT is measured in degrees Kelvin (K): warm light is about 2700 K, neutral white about 4000 K, and cool white is 5000K or more (Figure 7). Luminus LEDs are available across this full range of CCTs from 1800 K to 8000 K for use in a wide variety of lighting applications.

Spectral Power Distribution (SPD)
Spectral power distributions quantify the power of a light source as a function of wavelength. In other words, SPD describes how much optical power the light source emits for each wavelength band measured by a spectrometer. The radiant flux characteristics of light sources are typically represented with an SPD graph, where wavelengths (in nanometers, nm) are on the X-axis. Spectral power (measured in Watts per nanometer, W/nm) is distributed on the Y-axis, commonly normalized as an arbitrary unit such as intensity, as in Figure 8, or normalized intensity/relative sensitivity in Figure 10. The measured SPD is combined with a mathematical representation of eye response to calculate perceived color.
Note that SPD is not equivalent to CCT. CCT characterizes how humans perceive the color tonality of a light source; SPD captures the entire spectrum of wavelengths that a light outputs, including some that are invisible to the human eye, but which may still have circadian effects. You can understand CCT as an approximation of spectral content [4].
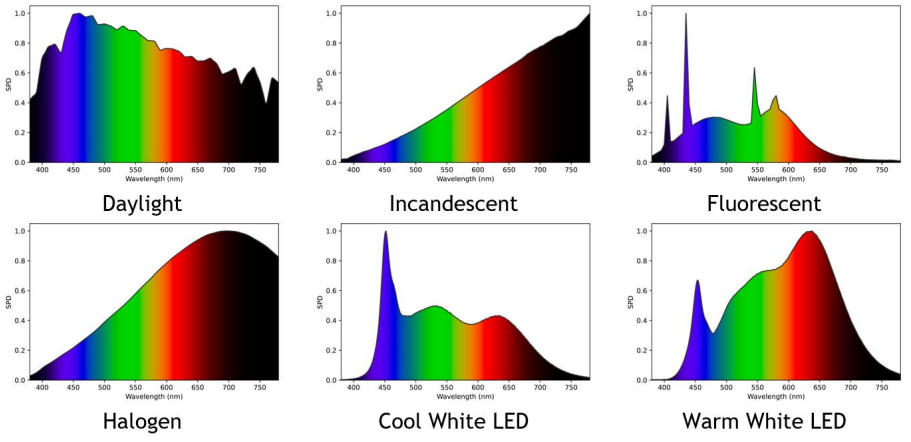
The Color Rendering Index (CRI)
It is important to know the color (CCT) of a light source to understand its general effect on humans. But for lighting design applications it is also important to understand its impact on the perceived visual environment. The color rendering index (CRI) measures how accurately a light source illuminates (renders) color. Traditional CRI is based on the average accuracy of how the source displays using defined color reference samples.
On a scale up to 100 (excellent), light sources with a CRI score of 50+ are considered usable depending on the application. The CRI of premium LEDs range from 80 to 95 minimums. While CRI is often reported as a single number, it represents the average score of a light source’s performance in rendering a set of standardized color samples. Traditionally CRI uses eight core-samples plus seven supplemental samples, each called Rx. For example, R9 is a deep red color commonly referenced as a key color quality indicator for many applications.
More recently, the Illumating Engineering Society (IES) released a new standard for assessing the color of LED light fixtures, TM 30-18, which has 99 individual color samples (replacing the 15 traditional CRI samples). More information and data regarding TM-30-18 color rendering for Luminus products is available on request. Contact: techsupport@luminus.com.
The Cyan Gap
Standard white LEDs typically exhibit a “dip” in the cyan region of the visible light spectrum. This dip, also called the cyan gap, can reduce the quality of light produced by these LEDs and make them less effective for applications requiring full-spectrum lighting.
For example, let’s consider an office lighting design that should promote alertness and productivity, which requires a target amount of cyan wavelength. If the available LEDs have low levels of cyan, then designers must use many more LEDs to reach the threshold of cyan needed to reach the target. This “overlighting” would flood a space with excess light in wavelengths that don’t contribute to circadian function while creating an uncomfortably bright environment.
One technology achievement on the road to HCL that addresses the cyan gap is the Luminus PerfectWhite™ COB LED (Figure 9). Perfect White eliminates the cyan gap and reduces potentially harmful UV wavelengths, making these LEDs well suited for situations requiring alertness or for use in spaces such as art museums where those light wavelengths can damage objects over time.
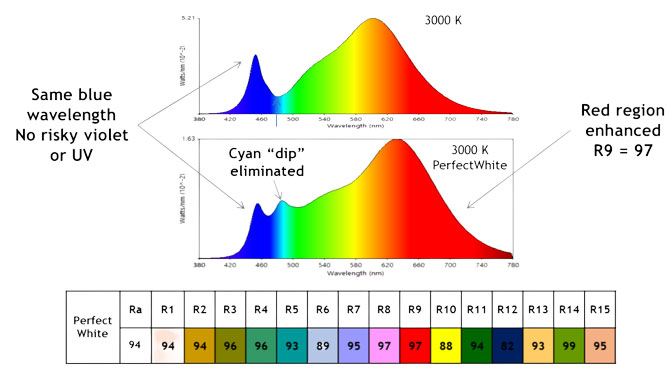
What Is Healthy Light?
The sun provides 6500 K light most of the day. This is the whitish and bluish light that suppresses melatonin production and boosts wakefulness. Melatonin, called “the sleep hormone,” is produced to signal the body to wind down for sleep; without it, we remain more alert.
Both the presence and color of light affect the body clock or circadian rhythm. This effect is known as melanopic vision, defined as the biological impact of light and how it affects production of the hormone melatonin. The naturally blue-rich spectrum of day light suppresses production of this hormone. As day draws to a close, dusk offers warmer orange and reddish tones and blue light recedes in the evening hours. In response, our bodies start to produce more melatonin to helps us fall asleep.
When our ancestors mastered fire, it brought more light into the evening and nighttime hours. However, because fire is a warm light that closely matches natural evening sunlight, it doesn’t have an adverse affect on natural sleep patterns.
The Melanopic Ratio
Photopic vision refers to what we see during the day and in a well-lit space, the visual impact of light (Figure 10). The Melanopic Ratio (MR)—which is also called the Melanopic/Photopic ratio, M/P ratio*, or MP ratio—quantifies the effect of light on circadian rhythm: how much a light source stimulates the human circadian system. Basically, the higher the MR ratio, the stronger the circadian effect of the light source, and therefore, the more strongly it promotes alertness and wakefulness.

Melanopic Ratio of Standard LED Light Sources
Candles, as we used for lighting in the past, have a CCT of around 1900 K, which is quite a warm light. More recently, LEDs have made it possible to fine-tune the CCT and color spectrum of lightbulbs and luminaires (Table 1). Artificial light sources can be designed at many different brightness levels (lux) and with different CCTs. Warmer light bulbs have a CCT around 2200 K and a low MR.
* NOTE: The Melanopic Ratio is also commonly referred to as the Melanopic/Photopic ratio or “M/P” ratio in the industry. CIE uses the term “MR” ratio. The melanopic/photopic ratio M/P should not be confused with mesopic luminance, also sometimes referred to as the “M/P ratio,” which describes outdoor lighting in relation to human night vision. In this white paper, we primarily use MR for the melanopic ratio to minimize confusion. They have fewer of the blue/cyan wavelengths and are best suited for nighttime relaxation and preparation for sleep.
By contrast, a light source with a cool CCT around 6500 K would tend to have a high MR with more blue/cyan, thus suppressing melatonin production. It would be ideal for daytime use and supporting alertness and productivity in schools, factories, offices, control rooms, etc. Figure 11 compares the color profile (SPD) of LEDs at different CCTs, and Figure 12 demonstrates how the light sources with different CCTs might look to a typical human viewer.
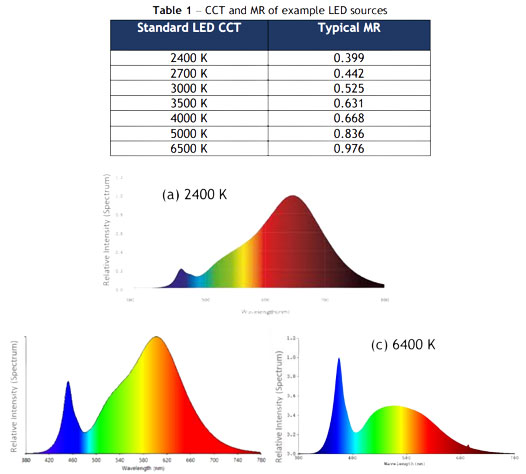

Human-Centric Lighting and How to Achieve It
How can architects, lighting designers, and building owners balance the need for artificial illumination indoors and at night with concerns for human well-being and productivity? The answer is human-centric lighting (HCL), a new, holistic approach developed in the last decade. HCL encompasses the science of circadian lighting to mimic the effect on our biological clock of natural daytime/nighttime light cycles, along with broader considerations for building occupants ’productivity, mood, and comfort.
Human-Centric Lighting 1.0: Using CCT in the Design
In essence, human-centric lighting uses the stimulating or relaxing effects of different color temperatures to advantage at different times of the day and in different contexts. For example, in the home, restaurants, or other venues where people spend their evenings and want to relax, warm white lights between 2200 K and 2700 K with a low blue content work well. LEDs in this CCT range have more blue wavelengths than candlelight but produce a warm white like that of an incandescent bulb, with a low MR. This CCT range is best for evening relaxation and preparation for sleep.
By contrast, cool white LEDs at around 6500 K works for daytime lighting in schools, offices, and other locations where alertness is essential. LEDs with this color temperature mimic the bluish cast of light on an overcast day, providing a high MR. They suppress melatonin to stimulate the circadian system to keep people awake, alert, and productive.
† The terminology for human perception of color temperature as “warm” (low CCT) and “cool” (high CCT) light, as shown in Figure 12, is the opposite of actual measurements of black-body color temperature, as shown in Figure 6. For black-body radiators, higher numbers mean higher temperatures (degrees Kelvin). For the purposes of this white paper, any mention of warm and cool light refers to the perceptual CCT, not black-body temperature, unless otherwise noted.
The drawback to this approach is that most people consider very cool white lighting to be harsh and uncomfortable. For this reason, lighting designers often aim for 4000 K in these environments, sacrificing some of the daylight benefits.
However, Luminus Salud LEDs offer a new solution. These LEDs have a high cyan content, and a high MR combined with more comfortable warm-light CCTs, providing a more optimal mixture for daytime indoor illumination. In addition to Luminus Salud, other technologies such as PerfectWhite and CCT tunable chips with full-spectrum color rendering have proven effective tools for meeting the latest HCL design standards and objectives. Further advancements will help lighting engineers and designers achieve optimal HCL—cost-effectively and without over lighting.
Human-Centric Lighting Basics (HCL 1.0)
Early understanding of the circadian effect of lighting led to some basic principles for achieving HCL, outlined below. More recently, science and LED technology advancements have enabled more sophisticated HCL approaches (see the Human Centric Lighting 2.0 section, below).
Daytime Lighting:
- Use cool ~6500 K CCT LEDs
- Cyan content in the spectrum
- High Melanopic Ratio
- Ideal for: schools, factories, offices, control rooms, etc.
Evening Lighting:
- Use a warm ~2200 – 2700 K CCT LEDs.
- Less cyan and blue present in the spectrum
- Low Melanopic Ratio
- Ideal for: evening and nighttime to promote relaxation and preparation for sleep
Human-Centric Lighting 2.0: Incorporating Melanopic Lux in the Design
These lighting quality parameters have become a new standard in architecture and design, but are still evolving. If HCL 1.0 means simply understanding the impact of CCT on humans and choosing cool or warm light fixtures appropriate to the purpose and activities of different indoor spaces, then incorporating melanopic lux as a factor in lighting layout designs could be considered the next level of HCL lighting—HCL 2.0.
New Standards for HCL Design
The WELL Building Standard®(WBS) is the first health-focused building standard to provide an evidence-based system for measuring, certifying, and monitoring the health impact of built environments. The WBS considers how construction and design can help the comfort, mood, sleep, and performance of people living and working in buildings.
The standard describes healthful light in Equivalent Melanopic Lux (EML). The EML formula stands beside other calculations for visible light as a method to quantify the biological (melanopic) effect of lighting on humans. The formula is weighted to emphasize the response of the retinal ganglion cells(ipRPGs), rather than the cones, which is the case with traditional lux.
An additional circadian cycle standard includes the Circadian Standard (CS) from Rensselaer Polytechnic Institute’s Lighting Enabled Systems & Applications (LESA) Center. Recently, Underwriters Laboratories (UL) decided to adopt the Rensselaer model.
Previously, industry standards accounted only for horizontal lux. Horizontal lux measures task lighting that shines on a flat surface such as a desktop or the floor. The new approach considers vertical lux, light that shines vertically and enters the pupil at eye level (Figure 13), which directly impacts our melanopic response. WBS defines “eye level” as 1.2 meters/4 feet above the floor. Lighting designers following the WBS must specify EML in both the vertical and horizontal plane, and suggests a daytime minimum of 200 EML. But, how much can a building’s over head lighting contribute to horizontal lux experienced by a human occupant? And, how does horizontal lux convert to vertical lux?
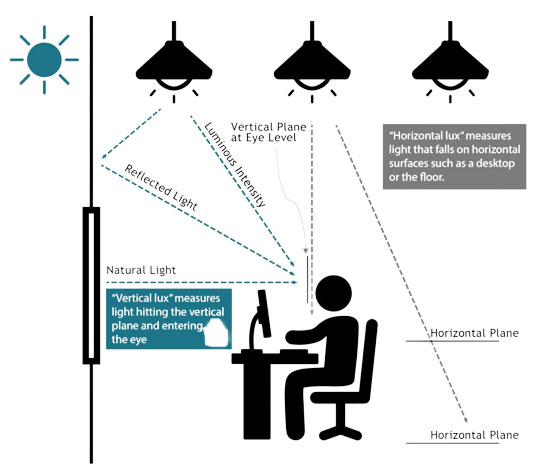
NOTE: Vertical lux and horizontal lux are common shorthand terms. Technically, vertical lux refers to the vertical photopic illuminance, Ev, and horizontal lux refers to the horizontal photopic illuminance, EH.
Calculating an HCL Design
There are several calculation methods and several steps to calculate a human-centric lighting scheme for any given interior environment. The process relies on concepts discussed above, including the Melanopic Ratio (MR) and Equivalent Melanopic Lux (EML). The process also uses data specific to the light/luminaire under consideration, such as its illuminance and spectral power distribution(SPD). Luminus recommends the following calculation method:
Step 1. Determine the light design criterion.
The WBS recommends designing for an EML ≥200 lux at human eye level (roughly 1.2 meters or 4 feet above the floor). It is not necessary to calculate a target EML before designing alighting scheme; for this first step, designers can simply follow the WBS guideline.
For reference, the procedure for calculating EML is:
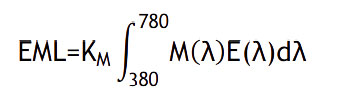
Where:
KM = 832.4 lm/W is the conversion factor of power (in watts, W) to luminous flux (in lumens, lm)
M(λ) is the normalized melanopic weighting function
E(λ) is the spectral irradiance at eye level, expressed as W/nm-m2
Step 2. Design a light plan.
Traditional lighting plans calculate lux only as horizontal lux. HCL designs that meet WBS must also consider vertical lux (vertical photopic illuminance). First, you must determine the luminous intensity distribution‡ of the lighting plan in a building space. A plan can use many different types of luminaires. For this example, we only use one type. Consider using lighting design software (such as DIALux) to calculate the spatial distribution in a building space and the vertical-to-horizontal illuminance ratio, which we will call RVH:
Vertical to horizontal ratio, RVH = EV/EH
Where:
EV = vertical photopic illuminance, or vertical lux
EH = horizontal photopic luminance, or horizontal lux
For an example, refer to the case study on page 23. We calculated the EV/EH ratio for the recessed downlight layout under consideration as 0.42. Thus, 500 horizontal lux has an associated 210 vertical lux.
Step 3. Select a light source SPD that provides the desired HCL attributes.
To start, you must determine the MR ratio, the circadian stimulation factor. There are at least four different methods for calculating the MR ratio of a light source [5], with different organizations recommending various methods. The methods are all related and can be equally effective depending on the specifics of a given lighting application.
Luminus recommends the method outlined in the WELL Building Standards. The method relies on the following formula, where spectral functions are normalized so that the maximum value is 1. To calculate the melanopic ratio:

Where:
P(λ) is the spectral power distribution of the light source
M(λ) is the normalized melanopic weighting function§ centered at 490 nm
V(λ) is the normalized photopic weighting function centered at 555 nm
K = 1.219, which is the conversion factor between the melanopic and photopic response to convert irradiance to lux
‡ Refer to Figure 5 for the definitions of luminance/illuminance, etc.
Step 4. Calculate EML.
To calculate the EML of the selected lighting system:
EML = EV × MR
Step 5. Compare results
Determine if the selected lighting system meets the circadian-effective lighting design criterion. Does the system EML meet the criterion (≥200 lux in this example) selected in Step 1?
Current Solutions for Achieving HCL
You can achieve a basic HCL lighting solution if you use the ecosystem of LED products already on the market. Technology for implementing CCT-specific lighting includes Luminus mid-power LEDs for distributed lighting and chip-on-board (COB) options for directional lighting. Dynamic COB products offer a simple choice of CCT ranges, either 3000 → 1800 K or 4000 → 2700 K. These products offer halogen-like or linear-style adjustment capabilities using a standard dimmer switch to control the CCT without lowering the light level.
An Innovative New Solution for HCL: Salud™
Ideally, human-centric lighting provides all the benefits of LED lighting, such as control, efficiency, and durability, with the latest technology to support HCL designs. The latest HCL principles call for warm CCTs with a high CRI and high MR. All these factors have come together in the world’s first LED with specified MR: the Salud™ Human Centric Mid-Power LED 3030-21C2.
The latest result of Luminus research and development, Salud LEDs, enable designers to meet melanopic lux targets and keep building occupants alert and awake without using 6500 K or overlighting the environment to reach the required level of melanopic lux. With full spectrum emission, high cyan content to ensure melatonin suppression (Figure 14), and accurate color rendering (minimum 90 CRI), Salud LEDs deliver high MR in a range of warmer CCTs that enable lighting that is comfortable and invigorating, with vibrant color rendition.
§ Refer to Figure 10 for comparison of the visual (photopic) and melanopic response curves.

Salud Product Benefits:
- Provides enhanced cyan spectrum without resorting to harsh blue 6500 K CCTs
- Enhanced cyan and no cyan gap
- Reduces glare
- Eliminates need for excessive lighting
- Saves power by reducing the number of luminaires needed to meet standards (refer to4000 K Office Case Study below)
- Is safer than sunlight, with no near-UV radiation
- Good color rendering (Ra 90+)
- Exceptional reds (high R9) for accurate color rendering, for example skin tones
- Available in 3000 K, 3500 K, 4000 K, and 5000 K versions

Table 2 − Salud Mid-Power LED Specifications**
** Luminus has Typical MR ratings for all of our white parts available on request (techsupport@luminus.com).
CASE STUDY: 4000 K Office Lighting Plan with Salud
For office settings, lighting designers typically use 4000 K, 80 CRI specifications to balance productivity and comfort for workers. A traditional 4000 K 80 CRI LED luminaire plan would require 40 recessed downlights to light a large open office cubicle space to provide 500 lux (horizontal lux)on each desk. For this example, Ev/EH has been determined to be 0.42.
However, the melanopic ratio (MR) of a traditional LED with 4000 K, 80 CRI is approximately 0.67.To achieve the EML standards with typical recessed downlights* if there’s a specification of 500 horizontal lux, calculate:
500 traditional lux (horizontal) x 0.67 = 335 melanopic lux (horizontal), then Melanopic lux (horizontal) x 0.42 = 140 melanopic lux (vertical)
Therefore, to reach 500 melanopic lux horizontal (or ~210 vertical), would require 1.5 times (50%)more light fixtures (60 downlights, instead of 40), increasing the cost. By contrast, using Salud 4000 K, 90 CRI LEDs with MR of 0.8 requires only 1.25 times (25%) more luminaires (e.g., 50 downlights). Using Salud 5000 K, 90 CRI with MR of 0.96, would require only 42 downlights to meet the EML standard requirement.
*Case study assumes each recessed downlight is engineered to deliver the same flux output (lumens).
Note that the conversion factor differs for each application, depending on the light source, wall reflectance, and other specifics of a given environment. For this example, we used the Rensselaer/LESA calculated value of 0.42. Lighting design software is often used to calculate the factor on a case-by-case basis. A typical downlight installation has Ev/EH close to 0.42 (for example, the UL24485 standard uses 0.45).
Conclusion
Humans are still learning how modern lighting affects our bodies and health. LED technology will continue to evolve to help architects, engineers, designers, and everyday consumers create indoor environments that boost human health and productivity. As awareness and adoption of HCL approaches rise, melanopic lux is becoming as important as CCT and CRI for evaluating lighting schemes. Our bodies need higher melanopic lux during the day; melanopic lux can be easily calculated using the lux level and MR of an LED spectrum.
Creating basic human centric lighting schemes requires selecting fixtures with the appropriate CCT,for example, Luminus CCT-tunable LEDs. For evenings, LEDs at 2200-2700 K help with relaxation as people prepare for sleep. For daytime, cooler CCT LEDs (e.g., 4000 K) promote alertness. The WELL Building Standard for MR is one of the leading guidelines to follow for creating HCL lighting schemes.
Previous LED technology was limited in its ability to meet WBS, however, requiring a very cool, bluish light at around 6500 K. But most people don’t enjoy working under that type of light. In fact, designers and architects usually design for around 4000 K. Typical LEDs have a cyan gap which requires designers to spend more and overlight spaces to ensure a comfortable environment while still achieving the minimum MR.
Salud LEDs are a breakthrough solution: because they fill the cyan gap, they enable you to meet the WBS without using uncomfortable super-cool CCT lights for indoor and office environments. Daytime LEDs can include a high MR with lower light levels and excellent color rendering. Salud helps lighting engineers to reach circadian standard targets that help optimize human productivity, comfort, and well being.
References
[1] American Academy of Sleep Medicine Board of Directors; Watson, N.F., et al., "Confronting Drowsy Driving; the American Academy of Sleep Medicine Perspective," Journal of Clinical Sleep Medicine, vol. 11, no. 11, pp. 1335-6, November 2015.
[2] Centers for Disease Control and Prevention: National Institute for Occupational Safety and Health (NIOSH), "The Color of Light Affects the Circadian Rhythms," [Online]. Available:https://www.cdc.gov/niosh/emres/longhourstraining/color.html. [Accessed 01 March 2021]
[3] J. K. H. D. Y. Dolijansky, "Working under daylight intensity lamp: an occupational risk for developing circadian rhythm sleep disorder?," Chronobiology International, vol. 22, no. 3, pp.597-605, 2005.
[4] WELL Building Standard, Feature 54, "Circadian Lighting Design," [Online]. Available:https://standard.wellcertified.com/light/circadian-lighting-design. [Accessed April 2021]
[5] J. Wood, "M/P ratios - Can we agree on how to calculate them?" 27 November 2019. [Online]. Available: https://www.ies.org. [Accessed 23 April 2021].